mirror of
https://github.com/LCTT/TranslateProject.git
synced 2025-01-25 23:11:02 +08:00
Translation Done
This commit is contained in:
parent
7b78440001
commit
b3d9169e3e
@ -1,622 +0,0 @@
|
||||
[Concurrent Servers: Part 3 - Event-driven][25]
|
||||
============================================================
|
||||
|
||||
GitFuture is Translating
|
||||
|
||||
This is part 3 of a series of posts on writing concurrent network servers. [Part 1][26] introduced the series with some building blocks, and [part 2 - Threads][27] discussed multiple threads as one viable approach for concurrency in the server.
|
||||
|
||||
Another common approach to achieve concurrency is called _event-driven programming_ , or alternatively _asynchronous_ programming [[1]][28]. The range of variations on this approach is very large, so we're going to start by covering the basics - using some of the fundamental APIs than form the base of most higher-level approaches. Future posts in the series will cover higher-level abstractions, as well as various hybrid approaches.
|
||||
|
||||
All posts in the series:
|
||||
|
||||
* [Part 1 - Introduction][12]
|
||||
|
||||
* [Part 2 - Threads][13]
|
||||
|
||||
* [Part 3 - Event-driven][14]
|
||||
|
||||
### Blocking vs. nonblocking I/O
|
||||
|
||||
As an introduction to the topic, let's talk about the difference between blocking and nonblocking I/O. Blocking I/O is easier to undestand, since this is the "normal" way we're used to I/O APIs working. While receiving data from a socket, a call to `recv` _blocks_ until some data is received from the peer connected to the other side of the socket. This is precisely the issue with the sequential server of part 1.
|
||||
|
||||
So blocking I/O has an inherent performance problem. We saw one way to tackle this problem in part 2, using multiple threads. As long as one thread is blocked on I/O, other threads can continue using the CPU. In fact, blocking I/O is usually very efficient on resource usage while the thread is waiting - the thread is put to sleep by the OS and only wakes up when whatever it was waiting for is available.
|
||||
|
||||
_Nonblocking_ I/O is a different approach. When a socket is set to nonblocking mode, a call to `recv` (and to `send`, but let's just focus on receiving here) will always return very quickly, even if there's no data to receive. In this case, it will return a special error status [[2]][15] notifying the caller that there's no data to receive at this time. The caller can then go do something else, or try to call `recv` again.
|
||||
|
||||
The difference between blocking and nonblocking `recv` is easiest to demonstrate with a simple code sample. Here's a small program that listens on a socket, continuously blocking on `recv`; when `recv` returns data, the program just reports how many bytes were received [[3]][16]:
|
||||
|
||||
```
|
||||
int main(int argc, const char** argv) {
|
||||
setvbuf(stdout, NULL, _IONBF, 0);
|
||||
|
||||
int portnum = 9988;
|
||||
if (argc >= 2) {
|
||||
portnum = atoi(argv[1]);
|
||||
}
|
||||
printf("Listening on port %d\n", portnum);
|
||||
|
||||
int sockfd = listen_inet_socket(portnum);
|
||||
struct sockaddr_in peer_addr;
|
||||
socklen_t peer_addr_len = sizeof(peer_addr);
|
||||
|
||||
int newsockfd = accept(sockfd, (struct sockaddr*)&peer_addr, &peer_addr_len);
|
||||
if (newsockfd < 0) {
|
||||
perror_die("ERROR on accept");
|
||||
}
|
||||
report_peer_connected(&peer_addr, peer_addr_len);
|
||||
|
||||
while (1) {
|
||||
uint8_t buf[1024];
|
||||
printf("Calling recv...\n");
|
||||
int len = recv(newsockfd, buf, sizeof buf, 0);
|
||||
if (len < 0) {
|
||||
perror_die("recv");
|
||||
} else if (len == 0) {
|
||||
printf("Peer disconnected; I'm done.\n");
|
||||
break;
|
||||
}
|
||||
printf("recv returned %d bytes\n", len);
|
||||
}
|
||||
|
||||
close(newsockfd);
|
||||
close(sockfd);
|
||||
|
||||
return 0;
|
||||
}
|
||||
```
|
||||
|
||||
The main loop repeatedly calls `recv` and reports what it returned (recall that `recv` returns 0 when the peer has disconnected). To try it out, we'll run this program in one terminal, and in a separate terminal connect to it with `nc`, sending a couple of short lines, separated by a delay of a couple of seconds:
|
||||
|
||||
```
|
||||
$ nc localhost 9988
|
||||
hello # wait for 2 seconds after typing this
|
||||
socket world
|
||||
^D # to end the connection>
|
||||
```
|
||||
|
||||
The listening program will print the following:
|
||||
|
||||
```
|
||||
$ ./blocking-listener 9988
|
||||
Listening on port 9988
|
||||
peer (localhost, 37284) connected
|
||||
Calling recv...
|
||||
recv returned 6 bytes
|
||||
Calling recv...
|
||||
recv returned 13 bytes
|
||||
Calling recv...
|
||||
Peer disconnected; I'm done.
|
||||
```
|
||||
|
||||
Now let's try a nonblocking version of the same listening program. Here it is:
|
||||
|
||||
```
|
||||
int main(int argc, const char** argv) {
|
||||
setvbuf(stdout, NULL, _IONBF, 0);
|
||||
|
||||
int portnum = 9988;
|
||||
if (argc >= 2) {
|
||||
portnum = atoi(argv[1]);
|
||||
}
|
||||
printf("Listening on port %d\n", portnum);
|
||||
|
||||
int sockfd = listen_inet_socket(portnum);
|
||||
struct sockaddr_in peer_addr;
|
||||
socklen_t peer_addr_len = sizeof(peer_addr);
|
||||
|
||||
int newsockfd = accept(sockfd, (struct sockaddr*)&peer_addr, &peer_addr_len);
|
||||
if (newsockfd < 0) {
|
||||
perror_die("ERROR on accept");
|
||||
}
|
||||
report_peer_connected(&peer_addr, peer_addr_len);
|
||||
|
||||
// Set nonblocking mode on the socket.
|
||||
int flags = fcntl(newsockfd, F_GETFL, 0);
|
||||
if (flags == -1) {
|
||||
perror_die("fcntl F_GETFL");
|
||||
}
|
||||
|
||||
if (fcntl(newsockfd, F_SETFL, flags | O_NONBLOCK) == -1) {
|
||||
perror_die("fcntl F_SETFL O_NONBLOCK");
|
||||
}
|
||||
|
||||
while (1) {
|
||||
uint8_t buf[1024];
|
||||
printf("Calling recv...\n");
|
||||
int len = recv(newsockfd, buf, sizeof buf, 0);
|
||||
if (len < 0) {
|
||||
if (errno == EAGAIN || errno == EWOULDBLOCK) {
|
||||
usleep(200 * 1000);
|
||||
continue;
|
||||
}
|
||||
perror_die("recv");
|
||||
} else if (len == 0) {
|
||||
printf("Peer disconnected; I'm done.\n");
|
||||
break;
|
||||
}
|
||||
printf("recv returned %d bytes\n", len);
|
||||
}
|
||||
|
||||
close(newsockfd);
|
||||
close(sockfd);
|
||||
|
||||
return 0;
|
||||
}
|
||||
```
|
||||
|
||||
A couple of notable differences from the blocking version:
|
||||
|
||||
1. The `newsockfd` socket returned by `accept` is set to nonblocking mode by calling `fcntl`.
|
||||
|
||||
2. When examining the return status of `recv`, we check whether `errno` is set to a value saying that no data is available for receiving. In this case we just sleep for 200 milliseconds and continue to the next iteration of the loop.
|
||||
|
||||
The same expermient with `nc` yields the following printout from this nonblocking listener:
|
||||
|
||||
```
|
||||
$ ./nonblocking-listener 9988
|
||||
Listening on port 9988
|
||||
peer (localhost, 37288) connected
|
||||
Calling recv...
|
||||
Calling recv...
|
||||
Calling recv...
|
||||
Calling recv...
|
||||
Calling recv...
|
||||
Calling recv...
|
||||
Calling recv...
|
||||
Calling recv...
|
||||
Calling recv...
|
||||
recv returned 6 bytes
|
||||
Calling recv...
|
||||
Calling recv...
|
||||
Calling recv...
|
||||
Calling recv...
|
||||
Calling recv...
|
||||
Calling recv...
|
||||
Calling recv...
|
||||
Calling recv...
|
||||
Calling recv...
|
||||
Calling recv...
|
||||
Calling recv...
|
||||
recv returned 13 bytes
|
||||
Calling recv...
|
||||
Calling recv...
|
||||
Calling recv...
|
||||
Peer disconnected; I'm done.
|
||||
```
|
||||
|
||||
As an exercise, add a timestamp to the printouts and convince yourself that the total time elapsed between fruitful calls to `recv` is more or less the delay in typing the lines into `nc` (rounded to the next 200 ms).
|
||||
|
||||
So there we have it - using nonblocking `recv` makes it possible for the listener the check in with the socket, and regain control if no data is available yet. Another word to describe this in the domain of programming is _polling_ - the main program periodically polls the socket for its readiness.
|
||||
|
||||
It may seem like a potential solution to the sequential serving issue. Nonblocking `recv` makes it possible to work with multiple sockets simulatenously, polling them for data and only handling those that have new data. This is true - concurrent servers _could_ be written this way; but in reality they don't, because the polling approach scales very poorly.
|
||||
|
||||
First, the 200 ms delay I introduced in the code above is nice for the demonstration (the listener prints only a few lines of "Calling recv..." between my typing into `nc` as opposed to thousands), but it also incurs a delay of up to 200 ms to the server's response time, which is almost certainly undesirable. In real programs the delay would have to be much shorter, and the shorter the sleep, the more CPU the process consumes. These are cycles consumed for just waiting, which isn't great, especially on mobile devices where power matters.
|
||||
|
||||
But the bigger problem happens when we actually have to work with multiple sockets this way. Imagine this listener is handling 1000 clients concurrently. This means that in every loop iteration, it has to do a nonblocking `recv` on _each and every one of those 1000 sockets_ , looking for one which has data ready. This is terribly inefficient, and severely limits the number of clients this server can handle concurrently. There's a catch-22 here: the longer we wait between polls, the less responsive the server is; the shorter we wait, the more CPU resources we burn on useless polling.
|
||||
|
||||
Frankly, all this polling also feels like useless work. Surely somewhere in the OS it is known which socket is actually ready with data, so we don't have to scan all of them. Indeed, it is, and the rest of this post will showcase a couple of APIs that let us handle multiple clients much more gracefully.
|
||||
|
||||
### select
|
||||
|
||||
The `select` system call is a portable (POSIX), venerable part of the standard Unix API. It was designed precisely for the problem described towards the end of the previous section - to allow a single thread to "watch" a non-trivial number of file descriptors [[4]][17] for changes, without needlessly spinning in a polling loop. I don't plan to include a comprehensive tutorial for `select` in this post - there are many websites and book chapters for that - but I will describe its API in the context of the problem we're trying to solve, and will present a fairly complete example.
|
||||
|
||||
`select` enables _I/O multiplexing_ - monitoring multiple file descriptors to see if I/O is possible on any of them.
|
||||
|
||||
```
|
||||
int select(int nfds, fd_set *readfds, fd_set *writefds,
|
||||
fd_set *exceptfds, struct timeval *timeout);
|
||||
```
|
||||
|
||||
`readfds` points to a buffer of file descriptors we're watching for read events; `fd_set` is an opaque data structure users manipulate using `FD_*` macros. `writefds` is the same for write events. `nfds` is the highest file descriptor number (file descriptors are just integers) in the watched buffers. `timeout` lets the user specify how long `select` should block waiting for one of the file descriptors to be ready (`timeout == NULL` means block indefinitely). I'll ignore `exceptfds` for now.
|
||||
|
||||
The contract of calling `select` is as follows:
|
||||
|
||||
1. Prior to the call, the user has to create `fd_set` instances for all the different kinds of descriptors to watch. If we want to watch for both read events and write events, both `readfds` and `writefds` should be created and populated.
|
||||
|
||||
2. The user uses `FD_SET` to set specific descriptors to watch in the set. For example, if we want to watch descriptors 2, 7 and 10 for read events, we call `FD_SET` three times on `readfds`, once for each of 2, 7 and 10.
|
||||
|
||||
3. `select` is called.
|
||||
|
||||
4. When `select` returns (let's ignore timeouts for now), it says how many descriptors in the sets passed to it are ready. It also modifies the `readfds` and `writefds` sets to mark only those descriptors that are ready. All the other descriptors are cleared.
|
||||
|
||||
5. At this point the user has to iterate over `readfds` and `writefds` to find which descriptors are ready (using `FD_ISSET`).
|
||||
|
||||
As a complete example, I've reimplemented our protocol in a concurrent server that uses `select`. The [full code is here][18]; what follows is some highlights from the code, with explanations. Warning: this code sample is fairly substantial - so feel free to skip it on first reading if you're short on time.
|
||||
|
||||
### A concurrent server using select
|
||||
|
||||
Using an I/O multiplexing API like `select` imposes certain constraints on the design of our server; these may not be immediately obvious, but are worth discussing since they are key to understanding what event-driven programming is all about.
|
||||
|
||||
Most importantly, always keep in mind that such an approach is, in its core, single-threaded [[5]][19]. The server really is just doing _one thing at a time_ . Since we want to handle multiple clients concurrently, we'll have to structure the code in an unusual way.
|
||||
|
||||
First, let's talk about the main loop. How would that look? To answer this question let's imagine our server during a flurry of activity - what should it watch for? Two kinds of socket activities:
|
||||
|
||||
1. New clients trying to connect. These clients should be `accept`-ed.
|
||||
|
||||
2. Existing client sending data. This data has to go through the usual protocol described in [part 1][11], with perhaps some data being sent back.
|
||||
|
||||
Even though these two activities are somewhat different in nature, we'll have to mix them into the same loop, because there can only be one main loop. Our loop will revolve around calls to `select`. This `select` call will watch for the two kinds of events described above.
|
||||
|
||||
Here's the part of the code that sets up the file descriptor sets and kicks off the main loop with a call to `select`:
|
||||
|
||||
```
|
||||
// The "master" sets are owned by the loop, tracking which FDs we want to
|
||||
// monitor for reading and which FDs we want to monitor for writing.
|
||||
fd_set readfds_master;
|
||||
FD_ZERO(&readfds_master);
|
||||
fd_set writefds_master;
|
||||
FD_ZERO(&writefds_master);
|
||||
|
||||
// The listenting socket is always monitored for read, to detect when new
|
||||
// peer connections are incoming.
|
||||
FD_SET(listener_sockfd, &readfds_master);
|
||||
|
||||
// For more efficiency, fdset_max tracks the maximal FD seen so far; this
|
||||
// makes it unnecessary for select to iterate all the way to FD_SETSIZE on
|
||||
// every call.
|
||||
int fdset_max = listener_sockfd;
|
||||
|
||||
while (1) {
|
||||
// select() modifies the fd_sets passed to it, so we have to pass in copies.
|
||||
fd_set readfds = readfds_master;
|
||||
fd_set writefds = writefds_master;
|
||||
|
||||
int nready = select(fdset_max + 1, &readfds, &writefds, NULL, NULL);
|
||||
if (nready < 0) {
|
||||
perror_die("select");
|
||||
}
|
||||
...
|
||||
```
|
||||
|
||||
A couple of points of interest here:
|
||||
|
||||
1. Since every call to `select` overwrites the sets given to the function, the caller has to maintain a "master" set to keep track of all the active sockets it monitors across loop iterations.
|
||||
|
||||
2. Note how, initially, the only socket we care about is `listener_sockfd`, which is the original socket on which the server accepts new clients.
|
||||
|
||||
3. The return value of `select` is the number of descriptors that are ready among those in the sets passed as arguments. The sets are modified by `select` to mark ready descriptors. The next step is iterating over the descriptors.
|
||||
|
||||
```
|
||||
...
|
||||
for (int fd = 0; fd <= fdset_max && nready > 0; fd++) {
|
||||
// Check if this fd became readable.
|
||||
if (FD_ISSET(fd, &readfds)) {
|
||||
nready--;
|
||||
|
||||
if (fd == listener_sockfd) {
|
||||
// The listening socket is ready; this means a new peer is connecting.
|
||||
...
|
||||
} else {
|
||||
fd_status_t status = on_peer_ready_recv(fd);
|
||||
if (status.want_read) {
|
||||
FD_SET(fd, &readfds_master);
|
||||
} else {
|
||||
FD_CLR(fd, &readfds_master);
|
||||
}
|
||||
if (status.want_write) {
|
||||
FD_SET(fd, &writefds_master);
|
||||
} else {
|
||||
FD_CLR(fd, &writefds_master);
|
||||
}
|
||||
if (!status.want_read && !status.want_write) {
|
||||
printf("socket %d closing\n", fd);
|
||||
close(fd);
|
||||
}
|
||||
}
|
||||
```
|
||||
|
||||
This part of the loop checks the _readable_ descriptors. Let's skip the listener socket (for the full scoop - [read the code][20]) and see what happens when one of the client sockets is ready. When this happens, we call a _callback_ function named `on_peer_ready_recv` with the file descriptor for the socket. This call means the client connected to that socket sent some data and a call to `recv` on the socket isn't expected to block [[6]][21]. This callback returns a struct of type `fd_status_t`:
|
||||
|
||||
```
|
||||
typedef struct {
|
||||
bool want_read;
|
||||
bool want_write;
|
||||
} fd_status_t;
|
||||
```
|
||||
|
||||
Which tells the main loop whether the socket should be watched for read events, write events, or both. The code above shows how `FD_SET` and `FD_CLR` are called on the appropriate descriptor sets accordingly. The code for a descriptor being ready for writing in the main loop is similar, except that the callback it invokes is called `on_peer_ready_send`.
|
||||
|
||||
Now it's time to look at the code for the callback itself:
|
||||
|
||||
```
|
||||
typedef enum { INITIAL_ACK, WAIT_FOR_MSG, IN_MSG } ProcessingState;
|
||||
|
||||
#define SENDBUF_SIZE 1024
|
||||
|
||||
typedef struct {
|
||||
ProcessingState state;
|
||||
|
||||
// sendbuf contains data the server has to send back to the client. The
|
||||
// on_peer_ready_recv handler populates this buffer, and on_peer_ready_send
|
||||
// drains it. sendbuf_end points to the last valid byte in the buffer, and
|
||||
// sendptr at the next byte to send.
|
||||
uint8_t sendbuf[SENDBUF_SIZE];
|
||||
int sendbuf_end;
|
||||
int sendptr;
|
||||
} peer_state_t;
|
||||
|
||||
// Each peer is globally identified by the file descriptor (fd) it's connected
|
||||
// on. As long as the peer is connected, the fd is uqique to it. When a peer
|
||||
// disconnects, a new peer may connect and get the same fd. on_peer_connected
|
||||
// should initialize the state properly to remove any trace of the old peer on
|
||||
// the same fd.
|
||||
peer_state_t global_state[MAXFDS];
|
||||
|
||||
fd_status_t on_peer_ready_recv(int sockfd) {
|
||||
assert(sockfd < MAXFDs);
|
||||
peer_state_t* peerstate = &global_state[sockfd];
|
||||
|
||||
if (peerstate->state == INITIAL_ACK ||
|
||||
peerstate->sendptr < peerstate->sendbuf_end) {
|
||||
// Until the initial ACK has been sent to the peer, there's nothing we
|
||||
// want to receive. Also, wait until all data staged for sending is sent to
|
||||
// receive more data.
|
||||
return fd_status_W;
|
||||
}
|
||||
|
||||
uint8_t buf[1024];
|
||||
int nbytes = recv(sockfd, buf, sizeof buf, 0);
|
||||
if (nbytes == 0) {
|
||||
// The peer disconnected.
|
||||
return fd_status_NORW;
|
||||
} else if (nbytes < 0) {
|
||||
if (errno == EAGAIN || errno == EWOULDBLOCK) {
|
||||
// The socket is not *really* ready for recv; wait until it is.
|
||||
return fd_status_R;
|
||||
} else {
|
||||
perror_die("recv");
|
||||
}
|
||||
}
|
||||
bool ready_to_send = false;
|
||||
for (int i = 0; i < nbytes; ++i) {
|
||||
switch (peerstate->state) {
|
||||
case INITIAL_ACK:
|
||||
assert(0 && "can't reach here");
|
||||
break;
|
||||
case WAIT_FOR_MSG:
|
||||
if (buf[i] == '^') {
|
||||
peerstate->state = IN_MSG;
|
||||
}
|
||||
break;
|
||||
case IN_MSG:
|
||||
if (buf[i] == '$') {
|
||||
peerstate->state = WAIT_FOR_MSG;
|
||||
} else {
|
||||
assert(peerstate->sendbuf_end < SENDBUF_SIZE);
|
||||
peerstate->sendbuf[peerstate->sendbuf_end++] = buf[i] + 1;
|
||||
ready_to_send = true;
|
||||
}
|
||||
break;
|
||||
}
|
||||
}
|
||||
// Report reading readiness iff there's nothing to send to the peer as a
|
||||
// result of the latest recv.
|
||||
return (fd_status_t){.want_read = !ready_to_send,
|
||||
.want_write = ready_to_send};
|
||||
}
|
||||
```
|
||||
|
||||
A `peer_state_t` is the full state object used to represent a client connection between callback calls from the main loop. Since a callback is invoked on some partial data sent by the client, it cannot assume it will be able to communicate with the client continuously, and it has to run quickly without blocking. It never blocks because the socket is set to non-blocking mode and `recv` will always return quickly. Other than calling `recv`, all this handler does is manipulate the state - there are no additional calls that could potentially block.
|
||||
|
||||
An an exercise, can you figure out why this code needs an extra state? Our servers so far in the series managed with just two states, but this one needs three.
|
||||
|
||||
Let's also have a look at the "socket ready to send" callback:
|
||||
|
||||
```
|
||||
fd_status_t on_peer_ready_send(int sockfd) {
|
||||
assert(sockfd < MAXFDs);
|
||||
peer_state_t* peerstate = &global_state[sockfd];
|
||||
|
||||
if (peerstate->sendptr >= peerstate->sendbuf_end) {
|
||||
// Nothing to send.
|
||||
return fd_status_RW;
|
||||
}
|
||||
int sendlen = peerstate->sendbuf_end - peerstate->sendptr;
|
||||
int nsent = send(sockfd, peerstate->sendbuf, sendlen, 0);
|
||||
if (nsent == -1) {
|
||||
if (errno == EAGAIN || errno == EWOULDBLOCK) {
|
||||
return fd_status_W;
|
||||
} else {
|
||||
perror_die("send");
|
||||
}
|
||||
}
|
||||
if (nsent < sendlen) {
|
||||
peerstate->sendptr += nsent;
|
||||
return fd_status_W;
|
||||
} else {
|
||||
// Everything was sent successfully; reset the send queue.
|
||||
peerstate->sendptr = 0;
|
||||
peerstate->sendbuf_end = 0;
|
||||
|
||||
// Special-case state transition in if we were in INITIAL_ACK until now.
|
||||
if (peerstate->state == INITIAL_ACK) {
|
||||
peerstate->state = WAIT_FOR_MSG;
|
||||
}
|
||||
|
||||
return fd_status_R;
|
||||
}
|
||||
}
|
||||
```
|
||||
|
||||
Same here - the callback calls a non-blocking `send` and performs state manipulation. In asynchronous code, it's critical for callbacks to do their work quickly - any delay blocks the main loop from making progress, and thus blocks the whole server from handling other clients.
|
||||
|
||||
Let's once again repeat a run of the server with the script that connects 3 clients simultaneously. In one terminal window we'll run:
|
||||
|
||||
```
|
||||
$ ./select-server
|
||||
```
|
||||
|
||||
In another:
|
||||
|
||||
```
|
||||
$ python3.6 simple-client.py -n 3 localhost 9090
|
||||
INFO:2017-09-26 05:29:15,864:conn1 connected...
|
||||
INFO:2017-09-26 05:29:15,864:conn2 connected...
|
||||
INFO:2017-09-26 05:29:15,864:conn0 connected...
|
||||
INFO:2017-09-26 05:29:15,865:conn1 sending b'^abc$de^abte$f'
|
||||
INFO:2017-09-26 05:29:15,865:conn2 sending b'^abc$de^abte$f'
|
||||
INFO:2017-09-26 05:29:15,865:conn0 sending b'^abc$de^abte$f'
|
||||
INFO:2017-09-26 05:29:15,865:conn1 received b'bcdbcuf'
|
||||
INFO:2017-09-26 05:29:15,865:conn2 received b'bcdbcuf'
|
||||
INFO:2017-09-26 05:29:15,865:conn0 received b'bcdbcuf'
|
||||
INFO:2017-09-26 05:29:16,866:conn1 sending b'xyz^123'
|
||||
INFO:2017-09-26 05:29:16,867:conn0 sending b'xyz^123'
|
||||
INFO:2017-09-26 05:29:16,867:conn2 sending b'xyz^123'
|
||||
INFO:2017-09-26 05:29:16,867:conn1 received b'234'
|
||||
INFO:2017-09-26 05:29:16,868:conn0 received b'234'
|
||||
INFO:2017-09-26 05:29:16,868:conn2 received b'234'
|
||||
INFO:2017-09-26 05:29:17,868:conn1 sending b'25$^ab0000$abab'
|
||||
INFO:2017-09-26 05:29:17,869:conn1 received b'36bc1111'
|
||||
INFO:2017-09-26 05:29:17,869:conn0 sending b'25$^ab0000$abab'
|
||||
INFO:2017-09-26 05:29:17,870:conn0 received b'36bc1111'
|
||||
INFO:2017-09-26 05:29:17,870:conn2 sending b'25$^ab0000$abab'
|
||||
INFO:2017-09-26 05:29:17,870:conn2 received b'36bc1111'
|
||||
INFO:2017-09-26 05:29:18,069:conn1 disconnecting
|
||||
INFO:2017-09-26 05:29:18,070:conn0 disconnecting
|
||||
INFO:2017-09-26 05:29:18,070:conn2 disconnecting
|
||||
```
|
||||
|
||||
Similarly to the threaded case, there's no delay between clients - they are all handled concurrently. And yet, there are no threads in sight in `select-server`! The main loop _multiplexes_ all the clients by efficient polling of multiple sockets using `select`. Recall the sequential vs. multi-threaded client handling diagrams from [part 2][22]. For our `select-server`, the time flow for three clients looks something like this:
|
||||
|
||||
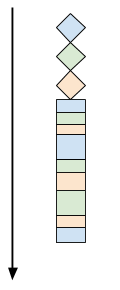
|
||||
|
||||
All clients are handled concurrently within the same thread, by multiplexing - doing some work for a client, switching to another, then another, then going back to the original client, etc. Note that there's no specific round-robin order here - the clients are handled when they send data to the server, which really depends on the client.
|
||||
|
||||
### Synchronous, asynchronous, event-driven, callback-based
|
||||
|
||||
The `select-server` code sample provides a good background for discussing just what is meant by "asynchronous" programming, and how it relates to event-driven and callback-based programming, because all these terms are common in the (rather inconsistent) discussion of concurrent servers.
|
||||
|
||||
Let's start with a quote from `select`'s man page:
|
||||
|
||||
> select, pselect, FD_CLR, FD_ISSET, FD_SET, FD_ZERO - synchronous I/O multiplexing
|
||||
|
||||
So `select` is for _synchronous_ multiplexing. But I've just presented a substantial code sample using `select` as an example of an _asynchronous_ server; what gives?
|
||||
|
||||
The answer is: it depends on your point of view. Synchronous is often used as a synonym for blocking, and the calls to `select` are, indeed, blocking. So are the calls to `send` and `recv` in the sequential and threaded servers presented in parts 1 and 2\. So it is fair to say that `select` is a _synchronous_ API. However, the server design emerging from the use of `select` is actually _asynchronous_ , or _callback-based_ , or _event-driven_ . Note that the `on_peer_*` functions presented in this post are callbacks; they should never block, and they get invoked due to network events. They can get partial data, and are expected to retain coherent state in-between invocations.
|
||||
|
||||
If you've done any amont of GUI programming in the past, all of this is very familiar. There's an "event loop" that's often entirely hidden in frameworks, and the application's "business logic" is built out of callbacks that get invoked by the event loop due to various events - user mouse clicks, menu selections, timers firing, data arriving on sockets, etc. The most ubiquitous model of programming these days is, of course, client-side Javascript, which is written as a bunch of callbacks invoked by user activity on a web page.
|
||||
|
||||
### The limitations of select
|
||||
|
||||
Using `select` for our first example of an asynchronous server makes sense to present the concept, and also because `select` is such an ubiquitous and portable API. But it also has some significant limitations that manifest when the number of watched file descriptors is very large:
|
||||
|
||||
1. Limited file descriptor set size.
|
||||
|
||||
2. Bad performance.
|
||||
|
||||
Let's start with the file descriptor size. `FD_SETSIZE` is a compile-time constant that's usually equal to 1024 on modern systems. It's hard-coded deep in the guts of `glibc`, and isn't easy to modify. It limits the number of file descriptors a `select` call can watch to 1024\. These days folks want to write servers that handle 10s of thousands of concurrent clients and more, so this problem is real. There are workarounds, but they aren't portable and aren't easy.
|
||||
|
||||
The bad performance issue is a bit more subtle, but still very serious. Note that when `select` returns, the information it provides to the caller is the number of "ready" descriptors, and the updated descriptor sets. The descriptor sets map from desrciptor to "ready/not ready" but they don't provide a way to iterate over all the ready descriptors efficiently. If there's only a single descriptor that is ready in the set, in the worst case the caller has to iterate over _the entire set_ to find it. This works OK when the number of descriptors watched is small, but if it gets to high numbers this overhead starts hurting [[7]][23].
|
||||
|
||||
For these reasons `select` has recently fallen out of favor for writing high-performance concurrent servers. Every popular OS has its own, non-portable APIs that permit users to write much more performant event loops; higher-level interfaces like frameworks and high-level languages usually wrap these APIs in a single portable interface.
|
||||
|
||||
### epoll
|
||||
|
||||
As an example, let's look at `epoll`, Linux's solution to the high-volume I/O event notification problem. The key to `epoll`'s efficiency is greater cooperation from the kernel. Instead of using a file descriptor set, `epoll_wait`fills a buffer with events that are currently ready. Only the ready events are added to the buffer, so there is no need to iterate over _all_ the currently watched file descriptors in the client. This changes the process of discovering which descriptors are ready from O(N) in `select`'s case to O(1).
|
||||
|
||||
A full presentation of the `epoll` API is not the goal here - there are plenty of online resources for that. As you may have guessed, though, I am going to write yet another version of our concurrent server - this time using `epoll` instead of `select`. The full code sample [is here][24]. In fact, since the vast majority of the code is the same as `select-server`, I'll only focus on the novelty - the use of `epoll` in the main loop:
|
||||
|
||||
```
|
||||
struct epoll_event accept_event;
|
||||
accept_event.data.fd = listener_sockfd;
|
||||
accept_event.events = EPOLLIN;
|
||||
if (epoll_ctl(epollfd, EPOLL_CTL_ADD, listener_sockfd, &accept_event) < 0) {
|
||||
perror_die("epoll_ctl EPOLL_CTL_ADD");
|
||||
}
|
||||
|
||||
struct epoll_event* events = calloc(MAXFDS, sizeof(struct epoll_event));
|
||||
if (events == NULL) {
|
||||
die("Unable to allocate memory for epoll_events");
|
||||
}
|
||||
|
||||
while (1) {
|
||||
int nready = epoll_wait(epollfd, events, MAXFDS, -1);
|
||||
for (int i = 0; i < nready; i++) {
|
||||
if (events[i].events & EPOLLERR) {
|
||||
perror_die("epoll_wait returned EPOLLERR");
|
||||
}
|
||||
|
||||
if (events[i].data.fd == listener_sockfd) {
|
||||
// The listening socket is ready; this means a new peer is connecting.
|
||||
...
|
||||
} else {
|
||||
// A peer socket is ready.
|
||||
if (events[i].events & EPOLLIN) {
|
||||
// Ready for reading.
|
||||
...
|
||||
} else if (events[i].events & EPOLLOUT) {
|
||||
// Ready for writing.
|
||||
...
|
||||
}
|
||||
}
|
||||
}
|
||||
}
|
||||
```
|
||||
|
||||
We start by configuring `epoll` with a call to `epoll_ctl`. In this case, the configuration amounts to adding the listening socket to the descriptors `epoll` is watching for us. We then allocate a buffer of ready events to pass to `epoll` for modification. The call to `epoll_wait` in the main loop is where the magic's at. It blocks until one of the watched descriptors is ready (or until a timeout expires), and returns the number of ready descriptors. This time, however, instead of blindly iterating over all the watched sets, we know that `epoll_write` populated the `events` buffer passed to it with the ready events, from 0 to `nready-1`, so we iterate only the strictly necessary number of times.
|
||||
|
||||
To reiterate this critical difference from `select`: if we're watching 1000 descriptors and two become ready, `epoll_waits` returns `nready=2` and populates the first two elements of the `events` buffer - so we only "iterate" over two descriptors. With `select` we'd still have to iterate over 1000 descriptors to find out which ones are ready. For this reason `epoll` scales much better than `select` for busy servers with many active sockets.
|
||||
|
||||
The rest of the code is straightforward, since we're already familiar with `select-server`. In fact, all the "business logic" of `epoll-server` is exactly the same as for `select-server` - the callbacks consist of the same code.
|
||||
|
||||
This similarity is tempting to exploit by abstracting away the event loop into a library/framework. I'm going to resist this itch, because so many great programmers succumbed to it in the past. Instead, in the next post we're going to look at `libuv` - one of the more popular event loop abstractions emerging recently. Libraries like `libuv` allow us to write concurrent asynchronous servers without worrying about the greasy details of the underlying system calls.
|
||||
|
||||
* * *
|
||||
|
||||
|
||||
[[1]][1] I tried enlightening myself on the actual semantic difference between the two by doing some web browsing and reading, but got a headache fairly quickly. There are many different opinions ranging from "they're the same thing", to "one is a subset of another" to "they're completely different things". When faced with such divergent views on the semantics, it's best to abandon the issue entirely, focusing instead on specific examples and use cases.
|
||||
|
||||
[[2]][2] POSIX mandates that this can be either `EAGAIN` or `EWOULDBLOCK`, and portable applications should check for both.
|
||||
|
||||
[[3]][3] Similarly to all C samples in this series, this code uses some helper utilities to set up listening sockets. The full code for these utilities lives in the `utils` module [in the repository][4].
|
||||
|
||||
[[4]][5] `select` is not a network/socket-specific function; it watches arbitrary file descriptors, which could be disk files, pipes, terminals, sockets or anything else Unix systems represent with file descriptors. In this post we're focusing on its uses for sockets, of course.
|
||||
|
||||
[[5]][6] There are ways to intermix event-driven programming with multiple threads, but I'll defer this discussion to later in the series.
|
||||
|
||||
|
||||
[[6]][7] Due to various non-trivial reasons it could _still_ block, even after `select` says it's ready. Therefore, all sockets opened by this server are set to nonblocking mode, and if the call to `recv` or `send` returns `EAGAIN` or `EWOULDBLOCK`, the callbacks just assumed no event really happened. Read the code sample comments for more details.
|
||||
|
||||
|
||||
[[7]][8] Note that this still isn't as bad as the asynchronous polling example presented earlier in the post. The polling has to happen _all the time_ , while `select` actually blocks until one or more sockets are ready for reading/writing; far less CPU time is wasted with `select` than with repeated polling.
|
||||
|
||||
|
||||
--------------------------------------------------------------------------------
|
||||
|
||||
via: https://eli.thegreenplace.net/2017/concurrent-servers-part-3-event-driven/
|
||||
|
||||
作者:[Eli Bendersky][a]
|
||||
译者:[译者ID](https://github.com/译者ID)
|
||||
校对:[校对者ID](https://github.com/校对者ID)
|
||||
|
||||
本文由 [LCTT](https://github.com/LCTT/TranslateProject) 原创编译,[Linux中国](https://linux.cn/) 荣誉推出
|
||||
|
||||
[a]:https://eli.thegreenplace.net/pages/about
|
||||
[1]:https://eli.thegreenplace.net/2017/concurrent-servers-part-3-event-driven/#id1
|
||||
[2]:https://eli.thegreenplace.net/2017/concurrent-servers-part-3-event-driven/#id3
|
||||
[3]:https://eli.thegreenplace.net/2017/concurrent-servers-part-3-event-driven/#id4
|
||||
[4]:https://github.com/eliben/code-for-blog/blob/master/2017/async-socket-server/utils.h
|
||||
[5]:https://eli.thegreenplace.net/2017/concurrent-servers-part-3-event-driven/#id5
|
||||
[6]:https://eli.thegreenplace.net/2017/concurrent-servers-part-3-event-driven/#id6
|
||||
[7]:https://eli.thegreenplace.net/2017/concurrent-servers-part-3-event-driven/#id8
|
||||
[8]:https://eli.thegreenplace.net/2017/concurrent-servers-part-3-event-driven/#id9
|
||||
[9]:https://eli.thegreenplace.net/tag/concurrency
|
||||
[10]:https://eli.thegreenplace.net/tag/c-c
|
||||
[11]:http://eli.thegreenplace.net/2017/concurrent-servers-part-1-introduction/
|
||||
[12]:http://eli.thegreenplace.net/2017/concurrent-servers-part-1-introduction/
|
||||
[13]:http://eli.thegreenplace.net/2017/concurrent-servers-part-2-threads/
|
||||
[14]:http://eli.thegreenplace.net/2017/concurrent-servers-part-3-event-driven/
|
||||
[15]:https://eli.thegreenplace.net/2017/concurrent-servers-part-3-event-driven/#id11
|
||||
[16]:https://eli.thegreenplace.net/2017/concurrent-servers-part-3-event-driven/#id12
|
||||
[17]:https://eli.thegreenplace.net/2017/concurrent-servers-part-3-event-driven/#id13
|
||||
[18]:https://github.com/eliben/code-for-blog/blob/master/2017/async-socket-server/select-server.c
|
||||
[19]:https://eli.thegreenplace.net/2017/concurrent-servers-part-3-event-driven/#id14
|
||||
[20]:https://github.com/eliben/code-for-blog/blob/master/2017/async-socket-server/select-server.c
|
||||
[21]:https://eli.thegreenplace.net/2017/concurrent-servers-part-3-event-driven/#id15
|
||||
[22]:http://eli.thegreenplace.net/2017/concurrent-servers-part-2-threads/
|
||||
[23]:https://eli.thegreenplace.net/2017/concurrent-servers-part-3-event-driven/#id16
|
||||
[24]:https://github.com/eliben/code-for-blog/blob/master/2017/async-socket-server/epoll-server.c
|
||||
[25]:https://eli.thegreenplace.net/2017/concurrent-servers-part-3-event-driven/
|
||||
[26]:http://eli.thegreenplace.net/2017/concurrent-servers-part-1-introduction/
|
||||
[27]:http://eli.thegreenplace.net/2017/concurrent-servers-part-2-threads/
|
||||
[28]:https://eli.thegreenplace.net/2017/concurrent-servers-part-3-event-driven/#id10
|
@ -0,0 +1,607 @@
|
||||
并发服务器(3) —— 事件驱动
|
||||
============================================================
|
||||
|
||||
这是《并发服务器》系列的第三节。[第一节][26] 介绍了阻塞式编程,[第二节 —— 线程][27] 探讨了多线程,将其作为一种可行的方法来实现服务器并发编程。
|
||||
|
||||
另一种常见的实现并发的方法叫做 _事件驱动编程_,也可以叫做 _异步_ 编程 [^注1][28]。这种方法变化万千,因此我们会从最基本的开始,使用一些基本的 APIs 而非从封装好的高级方法开始。本系列以后的文章会讲高层次抽象,还有各种混合的方法。
|
||||
|
||||
本系列的所有文章:
|
||||
|
||||
* [第一节 - 简介][12]
|
||||
* [第二节 - 线程][13]
|
||||
* [第三节 - 事件驱动][14]
|
||||
|
||||
### 阻塞式 vs. 非阻塞式 I/O
|
||||
|
||||
要介绍这个标题,我们先讲讲阻塞和非阻塞 I/O 的区别。阻塞式 I/O 更好理解,因为这是我们使用 I/O 相关 API 时的“标准”方式。从套接字接收数据的时候,调用 `recv` 函数会发生 _阻塞_,直到它从端口上接收到了来自另一端套接字的数据。这恰恰是第一部分讲到的顺序服务器的问题。
|
||||
|
||||
因此阻塞式 I/O 存在着固有的性能问题。第二节里我们讲过一种解决方法,就是用多线程。哪怕一个线程的 I/O 阻塞了,别的线程仍然可以使用 CPU 资源。实际上,阻塞 I/O 通常在利用资源方面非常高效,因为线程就等待着 —— 操作系统将线程变成休眠状态,只有满足了线程需要的条件才会被唤醒。
|
||||
|
||||
_非阻塞式_ I/O 是另一种思路。把套接字设成非阻塞模式时,调用 `recv` 时(还有 `send`,但是我们现在只考虑接收),函数返回地会很快,哪怕没有数据要接收。这时,就会返回一个特殊的错误状态 ^[注2][15] 来通知调用者,此时没有数据传进来。调用者可以去做其他的事情,或者尝试再次调用 `recv` 函数。
|
||||
|
||||
证明阻塞式和非阻塞式的 `recv` 区别的最好方式就是贴一段示例代码。这里有个监听套接字的小程序,一直在 `recv` 这里阻塞着;当 `recv` 返回了数据,程序就报告接收到了多少个字节 ^[注3][16]:
|
||||
|
||||
```
|
||||
int main(int argc, const char** argv) {
|
||||
setvbuf(stdout, NULL, _IONBF, 0);
|
||||
|
||||
int portnum = 9988;
|
||||
if (argc >= 2) {
|
||||
portnum = atoi(argv[1]);
|
||||
}
|
||||
printf("Listening on port %d\n", portnum);
|
||||
|
||||
int sockfd = listen_inet_socket(portnum);
|
||||
struct sockaddr_in peer_addr;
|
||||
socklen_t peer_addr_len = sizeof(peer_addr);
|
||||
|
||||
int newsockfd = accept(sockfd, (struct sockaddr*)&peer_addr, &peer_addr_len);
|
||||
if (newsockfd < 0) {
|
||||
perror_die("ERROR on accept");
|
||||
}
|
||||
report_peer_connected(&peer_addr, peer_addr_len);
|
||||
|
||||
while (1) {
|
||||
uint8_t buf[1024];
|
||||
printf("Calling recv...\n");
|
||||
int len = recv(newsockfd, buf, sizeof buf, 0);
|
||||
if (len < 0) {
|
||||
perror_die("recv");
|
||||
} else if (len == 0) {
|
||||
printf("Peer disconnected; I'm done.\n");
|
||||
break;
|
||||
}
|
||||
printf("recv returned %d bytes\n", len);
|
||||
}
|
||||
|
||||
close(newsockfd);
|
||||
close(sockfd);
|
||||
|
||||
return 0;
|
||||
}
|
||||
```
|
||||
|
||||
主循环重复调用 `recv` 并且报告它返回的字节数(记住 `recv` 返回 0 时,就是客户端断开连接了)。试着运行它,我们会在一个终端里运行这个程序,然后在另一个终端里用 `nc` 进行连接,发送一些字符,每次发送之间间隔几秒钟:
|
||||
|
||||
```
|
||||
$ nc localhost 9988
|
||||
hello # wait for 2 seconds after typing this
|
||||
socket world
|
||||
^D # to end the connection>
|
||||
```
|
||||
|
||||
The listening program will print the following:
|
||||
监听程序会输出以下内容:
|
||||
|
||||
```
|
||||
$ ./blocking-listener 9988
|
||||
Listening on port 9988
|
||||
peer (localhost, 37284) connected
|
||||
Calling recv...
|
||||
recv returned 6 bytes
|
||||
Calling recv...
|
||||
recv returned 13 bytes
|
||||
Calling recv...
|
||||
Peer disconnected; I'm done.
|
||||
```
|
||||
|
||||
现在试试非阻塞的监听程序的版本。这是代码:
|
||||
|
||||
```
|
||||
int main(int argc, const char** argv) {
|
||||
setvbuf(stdout, NULL, _IONBF, 0);
|
||||
|
||||
int portnum = 9988;
|
||||
if (argc >= 2) {
|
||||
portnum = atoi(argv[1]);
|
||||
}
|
||||
printf("Listening on port %d\n", portnum);
|
||||
|
||||
int sockfd = listen_inet_socket(portnum);
|
||||
struct sockaddr_in peer_addr;
|
||||
socklen_t peer_addr_len = sizeof(peer_addr);
|
||||
|
||||
int newsockfd = accept(sockfd, (struct sockaddr*)&peer_addr, &peer_addr_len);
|
||||
if (newsockfd < 0) {
|
||||
perror_die("ERROR on accept");
|
||||
}
|
||||
report_peer_connected(&peer_addr, peer_addr_len);
|
||||
|
||||
// 把套接字设成非阻塞模式
|
||||
int flags = fcntl(newsockfd, F_GETFL, 0);
|
||||
if (flags == -1) {
|
||||
perror_die("fcntl F_GETFL");
|
||||
}
|
||||
|
||||
if (fcntl(newsockfd, F_SETFL, flags | O_NONBLOCK) == -1) {
|
||||
perror_die("fcntl F_SETFL O_NONBLOCK");
|
||||
}
|
||||
|
||||
while (1) {
|
||||
uint8_t buf[1024];
|
||||
printf("Calling recv...\n");
|
||||
int len = recv(newsockfd, buf, sizeof buf, 0);
|
||||
if (len < 0) {
|
||||
if (errno == EAGAIN || errno == EWOULDBLOCK) {
|
||||
usleep(200 * 1000);
|
||||
continue;
|
||||
}
|
||||
perror_die("recv");
|
||||
} else if (len == 0) {
|
||||
printf("Peer disconnected; I'm done.\n");
|
||||
break;
|
||||
}
|
||||
printf("recv returned %d bytes\n", len);
|
||||
}
|
||||
|
||||
close(newsockfd);
|
||||
close(sockfd);
|
||||
|
||||
return 0;
|
||||
}
|
||||
```
|
||||
|
||||
这里与阻塞版本有些差异,值得注意:
|
||||
|
||||
1. `accept` 函数返回的 `newsocktfd` 套接字因调用了 `fcntl`, 被设置成非阻塞的模式。
|
||||
|
||||
2. 检查 `recv` 的返回状态时,我们对 `errno` 进行了检查,判断它是否被设置成表示没有可供接收的数据的状态。这时,我们仅仅是休眠了 200 毫秒然后进入到下一轮循环。
|
||||
|
||||
同样用 `nc` 进行测试,以下是非阻塞监听器的输出:
|
||||
|
||||
```
|
||||
$ ./nonblocking-listener 9988
|
||||
Listening on port 9988
|
||||
peer (localhost, 37288) connected
|
||||
Calling recv...
|
||||
Calling recv...
|
||||
Calling recv...
|
||||
Calling recv...
|
||||
Calling recv...
|
||||
Calling recv...
|
||||
Calling recv...
|
||||
Calling recv...
|
||||
Calling recv...
|
||||
recv returned 6 bytes
|
||||
Calling recv...
|
||||
Calling recv...
|
||||
Calling recv...
|
||||
Calling recv...
|
||||
Calling recv...
|
||||
Calling recv...
|
||||
Calling recv...
|
||||
Calling recv...
|
||||
Calling recv...
|
||||
Calling recv...
|
||||
Calling recv...
|
||||
recv returned 13 bytes
|
||||
Calling recv...
|
||||
Calling recv...
|
||||
Calling recv...
|
||||
Peer disconnected; I'm done.
|
||||
```
|
||||
|
||||
作为练习,给输出添加一个时间戳,确认调用 `recv` 得到结果之间花费的时间是比输入到 `nc` 中所用的多还是少(每一轮是 200 ms)。
|
||||
|
||||
这里就实现了使用非阻塞的 `recv` 让监听者检查套接字变为可能,并且在没有数据的时候重新获得控制权。换句话说,这就是 _polling(轮询)_ —— 主程序周期性的查询套接字以便读取数据。
|
||||
|
||||
对于顺序响应的问题,这似乎是个可行的方法。非阻塞的 `recv` 让同时与多个套接字通信变成可能,轮询这些套接字,仅当有新数据到来时才处理。就是这样,这种方式 _可以_ 用来写并发服务器;但实际上一般不这么做,因为轮询的方式很难扩展。
|
||||
|
||||
首先,我在代码中引入的 200 ms 延迟对于记录非常好(监听器在我输入 `nc` 之间只打印几行 “Calling recv...”,但实际上应该有上千行)。但它也增加了多达 200 ms 的服务器响应时间,这几乎是意料不到的。实际的程序中,延迟会低得多,休眠时间越短,进程占用的 CPU 资源就越多。有些时钟周期只是浪费在等待,这并不好,尤其是在移动设备上,这些设备的电量往往有限。
|
||||
|
||||
但是当我们实际这样来使用多个套接字的时候,更严重的问题出现了。想像下监听器正在同时处理 1000 个 客户端。这意味着每一个循环迭代里面,它都得为 _这 1000 个套接字中的每一个_ 执行一遍非阻塞的 `recv`,找到其中准备好了数据的那一个。这非常低效,并且极大的限制了服务器能够并发处理的客户端数。这里有个准则:每次轮询之间等待的间隔越久,服务器响应性越差;而等待的时间越少,CPU 在无用的轮询上耗费的资源越多。
|
||||
|
||||
讲真,所有的轮询都像是无用功。当然操作系统应该是知道哪个套接字是准备好了数据的,因此没必要逐个扫描。事实上,就是这样,接下来就会讲一些API,让我们可以更优雅地处理多个客户端。
|
||||
|
||||
### select
|
||||
|
||||
`select` 的系统调用是轻便的(POSIX),标准 Unix API 中常有的部分。它是为上一节最后一部分描述的问题而设计的 —— 允许一个线程可以监视许多文件描述符 ^[注4][17] 的变化,不用在轮询中执行不必要的代码。我并不打算在这里引入一个关于 `select` 的理解性的教程,有很多网站和书籍讲这个,但是在涉及到问题的相关内容时,我会介绍一下它的 API,然后再展示一个非常复杂的例子。
|
||||
|
||||
`select` 允许 _多路 I/O_,监视多个文件描述符,查看其中任何一个的 I/O 是否可用。
|
||||
|
||||
```
|
||||
int select(int nfds, fd_set *readfds, fd_set *writefds,
|
||||
fd_set *exceptfds, struct timeval *timeout);
|
||||
```
|
||||
|
||||
`readfds` 指向文件描述符的缓冲区,这个缓冲区被监视是否有读取事件;`fd_set` 是一个特殊的数据结构,用户使用 `FD_*` 宏进行操作。`writefds` 是针对写事件的。`nfds` 是监视的缓冲中最大的文件描述符数字(文件描述符就是整数)。`timeout` 可以让用户指定 `select` 应该阻塞多久,直到某个文件描述符准备好了(`timeout == NULL` 就是说一直阻塞着)。现在先跳过 `exceptfds`。
|
||||
|
||||
`select` 的调用过程如下:
|
||||
|
||||
1. 在调用之前,用户先要为所有不同种类的要监视的文件描述符创建 `fd_set` 实例。如果想要同时监视读取和写入事件,`readfds` 和 `writefds` 都要被创建并且引用。
|
||||
|
||||
2. 用户可以使用 `FD_SET` 来设置集合中想要监视的特殊描述符。例如,如果想要监视描述符 2、7 和 10 的读取事件,在 `readfds` 这里调用三次 `FD_SET`,分别设置 2、7 和 10。
|
||||
|
||||
3. `select` 被调用。
|
||||
|
||||
4. 当 `select` 返回时(现在先不管超时),就是说集合中有多少个文件描述符已经就绪了。它也修改 `readfds` 和 `writefds` 集合,来标记这些准备好的描述符。其它所有的描述符都会被清空。
|
||||
|
||||
5. 这时用户需要遍历 `readfds` 和 `writefds`,找到哪个描述符就绪了(使用 `FD_ISSET`)。
|
||||
|
||||
作为完整的例子,我在并发的服务器程序上使用 `select`,重新实现了我们之前的协议。[完整的代码在这里][18];接下来的是代码中的高亮,还有注释。警告:示例代码非常复杂,因此第一次看的时候,如果没有足够的时间,快速浏览也没有关系。
|
||||
|
||||
### 使用 select 的并发服务器
|
||||
|
||||
使用 I/O 的多发 API 诸如 `select` 会给我们服务器的设计带来一些限制;这不会马上显现出来,但这值得探讨,因为它们是理解事件驱动编程到底是什么的关键。
|
||||
|
||||
最重要的是,要记住这种方法本质上是单线程的 ^[注5][19]。服务器实际上在 _同一时刻只能做一件事_。因为我们想要同时处理多个客户端请求,我们需要换一种方式重构代码。
|
||||
|
||||
首先,让我们谈谈主循环。它看起来是什么样的呢?先让我们想象一下服务器有一堆任务,它应该监视哪些东西呢?两种类型的套接字活动:
|
||||
|
||||
1. 新客户端尝试连接。这些客户端应该被 `accept`。
|
||||
|
||||
2. 已连接的客户端发送数据。这个数据要用 [第一节][11] 中所讲到的协议进行传输,有可能会有一些数据要被回送给客户端。
|
||||
|
||||
尽管这两种活动在本质上有所区别,我们还是要把他们放在一个循环里,因为只能有一个主循环。循环会包含 `select` 的调用。这个 `select` 的调用会监视上述的两种活动。
|
||||
|
||||
这里是部分代码,设置了文件描述符集合,并在主循环里转到被调用的 `select` 部分。
|
||||
|
||||
```
|
||||
// “master” 集合存活在该循环中,跟踪我们想要监视的读取事件或写入事件的文件描述符(FD)。
|
||||
fd_set readfds_master;
|
||||
FD_ZERO(&readfds_master);
|
||||
fd_set writefds_master;
|
||||
FD_ZERO(&writefds_master);
|
||||
|
||||
// 监听的套接字一直被监视,用于读取数据,并监测到来的新的端点连接。
|
||||
FD_SET(listener_sockfd, &readfds_master);
|
||||
|
||||
// 要想更加高效,fdset_max 追踪当前已知最大的 FD;这使得每次调用时对 FD_SETSIZE 的迭代选择不是那么重要了。
|
||||
int fdset_max = listener_sockfd;
|
||||
|
||||
while (1) {
|
||||
// select() 会修改传递给它的 fd_sets,因此进行拷贝一下再传值。
|
||||
fd_set readfds = readfds_master;
|
||||
fd_set writefds = writefds_master;
|
||||
|
||||
int nready = select(fdset_max + 1, &readfds, &writefds, NULL, NULL);
|
||||
if (nready < 0) {
|
||||
perror_die("select");
|
||||
}
|
||||
...
|
||||
```
|
||||
|
||||
这里的一些要点:
|
||||
|
||||
1. 由于每次调用 `select` 都会重写传递给函数的集合,调用器就得维护一个 “master” 集合,在循环迭代中,保持对所监视的所有活跃的套接字的追踪。
|
||||
|
||||
2. 注意我们所关心的,最开始的唯一那个套接字是怎么变成 `listener_sockfd` 的,这就是最开始的套接字,服务器借此来接收新客户端的连接。
|
||||
|
||||
3. `select` 的返回值,是在作为参数传递的集合中,那些已经就绪的描述符的个数。`select` 修改这个集合,用来标记就绪的描述符。下一步是在这些描述符中进行迭代。
|
||||
|
||||
```
|
||||
...
|
||||
for (int fd = 0; fd <= fdset_max && nready > 0; fd++) {
|
||||
// 检查 fd 是否变成可读的
|
||||
if (FD_ISSET(fd, &readfds)) {
|
||||
nready--;
|
||||
|
||||
if (fd == listener_sockfd) {
|
||||
// 监听的套接字就绪了;这意味着有个新的客户端连接正在联系
|
||||
...
|
||||
} else {
|
||||
fd_status_t status = on_peer_ready_recv(fd);
|
||||
if (status.want_read) {
|
||||
FD_SET(fd, &readfds_master);
|
||||
} else {
|
||||
FD_CLR(fd, &readfds_master);
|
||||
}
|
||||
if (status.want_write) {
|
||||
FD_SET(fd, &writefds_master);
|
||||
} else {
|
||||
FD_CLR(fd, &writefds_master);
|
||||
}
|
||||
if (!status.want_read && !status.want_write) {
|
||||
printf("socket %d closing\n", fd);
|
||||
close(fd);
|
||||
}
|
||||
}
|
||||
```
|
||||
|
||||
这部分循环检查 _可读的_ 描述符。让我们跳过监听器套接字(要浏览所有内容,[看这个代码][20]) 然后看看当其中一个客户端准备好了之后会发生什么。出现了这种情况后,我们调用一个叫做 `on_peer_ready_recv` 的 _回调_ 函数,传入相应的文件描述符。这个调用意味着客户端连接到套接字上,发送某些数据,并且对套接字上 `recv` 的调用不会被阻塞 ^[注6][21]。这个回调函数返回结构体 `fd_status_t`。
|
||||
|
||||
```
|
||||
typedef struct {
|
||||
bool want_read;
|
||||
bool want_write;
|
||||
} fd_status_t;
|
||||
```
|
||||
|
||||
这个结构体告诉主循环,是否应该监视套接字的读取事件,写入事件,或者两者都监视。上述代码展示了 `FD_SET` 和 `FD_CLR` 是怎么在合适的描述符集合中被调用的。对于主循环中某个准备好了写入数据的描述符,代码是类似的,除了它所调用的回调函数,这个回调函数叫做 `on_peer_ready_send`。
|
||||
|
||||
现在来花点时间看看这个回调:
|
||||
|
||||
```
|
||||
typedef enum { INITIAL_ACK, WAIT_FOR_MSG, IN_MSG } ProcessingState;
|
||||
|
||||
#define SENDBUF_SIZE 1024
|
||||
|
||||
typedef struct {
|
||||
ProcessingState state;
|
||||
|
||||
// sendbuf 包含了服务器要返回给客户端的数据。on_peer_ready_recv 句柄填充这个缓冲,
|
||||
// on_peer_read_send 进行消耗。sendbuf_end 指向缓冲区的最后一个有效字节,
|
||||
// sendptr 指向下个字节
|
||||
uint8_t sendbuf[SENDBUF_SIZE];
|
||||
int sendbuf_end;
|
||||
int sendptr;
|
||||
} peer_state_t;
|
||||
|
||||
// 每一端都是通过它连接的文件描述符(fd)进行区分。只要客户端连接上了,fd 就是唯一的。
|
||||
// 当客户端断开连接,另一个客户端连接上就会获得相同的 fd。on_peer_connected 应该
|
||||
// 进行初始化,以便移除旧客户端在同一个 fd 上留下的东西。
|
||||
peer_state_t global_state[MAXFDS];
|
||||
|
||||
fd_status_t on_peer_ready_recv(int sockfd) {
|
||||
assert(sockfd < MAXFDs);
|
||||
peer_state_t* peerstate = &global_state[sockfd];
|
||||
|
||||
if (peerstate->state == INITIAL_ACK ||
|
||||
peerstate->sendptr < peerstate->sendbuf_end) {
|
||||
// 在初始的 ACK 被送到了客户端,就没有什么要接收的了。
|
||||
// 等所有待发送的数据都被发送之后接收更多的数据。
|
||||
return fd_status_W;
|
||||
}
|
||||
|
||||
uint8_t buf[1024];
|
||||
int nbytes = recv(sockfd, buf, sizeof buf, 0);
|
||||
if (nbytes == 0) {
|
||||
// 客户端断开连接
|
||||
return fd_status_NORW;
|
||||
} else if (nbytes < 0) {
|
||||
if (errno == EAGAIN || errno == EWOULDBLOCK) {
|
||||
// 套接字 *实际* 并没有准备好接收,等到它就绪。
|
||||
return fd_status_R;
|
||||
} else {
|
||||
perror_die("recv");
|
||||
}
|
||||
}
|
||||
bool ready_to_send = false;
|
||||
for (int i = 0; i < nbytes; ++i) {
|
||||
switch (peerstate->state) {
|
||||
case INITIAL_ACK:
|
||||
assert(0 && "can't reach here");
|
||||
break;
|
||||
case WAIT_FOR_MSG:
|
||||
if (buf[i] == '^') {
|
||||
peerstate->state = IN_MSG;
|
||||
}
|
||||
break;
|
||||
case IN_MSG:
|
||||
if (buf[i] == '$') {
|
||||
peerstate->state = WAIT_FOR_MSG;
|
||||
} else {
|
||||
assert(peerstate->sendbuf_end < SENDBUF_SIZE);
|
||||
peerstate->sendbuf[peerstate->sendbuf_end++] = buf[i] + 1;
|
||||
ready_to_send = true;
|
||||
}
|
||||
break;
|
||||
}
|
||||
}
|
||||
// 如果没有数据要发送给客户端,报告读取状态作为最后接收的结果。
|
||||
return (fd_status_t){.want_read = !ready_to_send,
|
||||
.want_write = ready_to_send};
|
||||
}
|
||||
```
|
||||
|
||||
`peer_state_t` 是全状态对象,用来表示在主循环中两次回调函数调用之间的客户端的连接。因为回调函数在客户端发送的某些数据时被调用,不能假设它能够不停地与客户端通信,并且它得运行得很快,不能被阻塞。因为套接字被设置成非阻塞模式,`recv` 会快速的返回。除了调用 `recv`, 这个句柄做的是处理状态,没有其它的调用,从而不会发生阻塞。
|
||||
|
||||
举个例子,你知道为什么这个代码需要一个额外的状态吗?这个系列中,我们的服务器目前只用到了两个状态,但是这个服务器程序需要三个状态。
|
||||
|
||||
来看看 “套接字准备好发送” 的回调函数:
|
||||
|
||||
```
|
||||
fd_status_t on_peer_ready_send(int sockfd) {
|
||||
assert(sockfd < MAXFDs);
|
||||
peer_state_t* peerstate = &global_state[sockfd];
|
||||
|
||||
if (peerstate->sendptr >= peerstate->sendbuf_end) {
|
||||
// 没有要发送的。
|
||||
return fd_status_RW;
|
||||
}
|
||||
int sendlen = peerstate->sendbuf_end - peerstate->sendptr;
|
||||
int nsent = send(sockfd, peerstate->sendbuf, sendlen, 0);
|
||||
if (nsent == -1) {
|
||||
if (errno == EAGAIN || errno == EWOULDBLOCK) {
|
||||
return fd_status_W;
|
||||
} else {
|
||||
perror_die("send");
|
||||
}
|
||||
}
|
||||
if (nsent < sendlen) {
|
||||
peerstate->sendptr += nsent;
|
||||
return fd_status_W;
|
||||
} else {
|
||||
// 所有东西都成功发送;重置发送队列。
|
||||
peerstate->sendptr = 0;
|
||||
peerstate->sendbuf_end = 0;
|
||||
|
||||
// 如果我们现在是处于特殊的 INITIAL_ACK 状态,就转变到其他状态。
|
||||
if (peerstate->state == INITIAL_ACK) {
|
||||
peerstate->state = WAIT_FOR_MSG;
|
||||
}
|
||||
|
||||
return fd_status_R;
|
||||
}
|
||||
}
|
||||
```
|
||||
|
||||
这里也一样,回调函数调用了一个非阻塞的 `send`,演示了状态管理。在异步代码中,回调函数执行的很快是受争议的,任何延迟都会阻塞主循环进行处理,因此也阻塞了整个服务器程序去处理其他的客户端。
|
||||
|
||||
用脚步再来运行这个服务器,同时连接 3 个客户端。在一个终端中我们运行下面的命令:
|
||||
|
||||
```
|
||||
$ ./select-server
|
||||
```
|
||||
|
||||
在另一个终端中:
|
||||
|
||||
```
|
||||
$ python3.6 simple-client.py -n 3 localhost 9090
|
||||
INFO:2017-09-26 05:29:15,864:conn1 connected...
|
||||
INFO:2017-09-26 05:29:15,864:conn2 connected...
|
||||
INFO:2017-09-26 05:29:15,864:conn0 connected...
|
||||
INFO:2017-09-26 05:29:15,865:conn1 sending b'^abc$de^abte$f'
|
||||
INFO:2017-09-26 05:29:15,865:conn2 sending b'^abc$de^abte$f'
|
||||
INFO:2017-09-26 05:29:15,865:conn0 sending b'^abc$de^abte$f'
|
||||
INFO:2017-09-26 05:29:15,865:conn1 received b'bcdbcuf'
|
||||
INFO:2017-09-26 05:29:15,865:conn2 received b'bcdbcuf'
|
||||
INFO:2017-09-26 05:29:15,865:conn0 received b'bcdbcuf'
|
||||
INFO:2017-09-26 05:29:16,866:conn1 sending b'xyz^123'
|
||||
INFO:2017-09-26 05:29:16,867:conn0 sending b'xyz^123'
|
||||
INFO:2017-09-26 05:29:16,867:conn2 sending b'xyz^123'
|
||||
INFO:2017-09-26 05:29:16,867:conn1 received b'234'
|
||||
INFO:2017-09-26 05:29:16,868:conn0 received b'234'
|
||||
INFO:2017-09-26 05:29:16,868:conn2 received b'234'
|
||||
INFO:2017-09-26 05:29:17,868:conn1 sending b'25$^ab0000$abab'
|
||||
INFO:2017-09-26 05:29:17,869:conn1 received b'36bc1111'
|
||||
INFO:2017-09-26 05:29:17,869:conn0 sending b'25$^ab0000$abab'
|
||||
INFO:2017-09-26 05:29:17,870:conn0 received b'36bc1111'
|
||||
INFO:2017-09-26 05:29:17,870:conn2 sending b'25$^ab0000$abab'
|
||||
INFO:2017-09-26 05:29:17,870:conn2 received b'36bc1111'
|
||||
INFO:2017-09-26 05:29:18,069:conn1 disconnecting
|
||||
INFO:2017-09-26 05:29:18,070:conn0 disconnecting
|
||||
INFO:2017-09-26 05:29:18,070:conn2 disconnecting
|
||||
```
|
||||
|
||||
和线程的情况相似,客户端之间没有延迟,他们被同时处理。而且在 `select-server` 也没有用线程!主循环 _多路_ 处理所有的客户端,通过高效使用 `select` 轮询多个套接字。回想下 [第二节中][22] 顺序的 vs 多线程的客户端处理过程的图片。对于我们的 `select-server`,三个客户端的处理流程像这样:
|
||||
|
||||
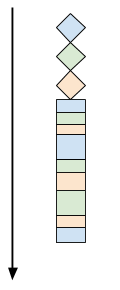
|
||||
|
||||
所有的客户端在同一个线程中同时被处理,通过乘积,做一点这个客户端的任务,然后切换到另一个,再切换到下一个,最后切换回到最开始的那个客户端。注意,这里没有什么循环调度,客户端在它们发送数据的时候被客户端处理,这实际上是受客户端左右的。
|
||||
|
||||
### 同步,异步,事件驱动,回调
|
||||
|
||||
`select-server` 示例代码为讨论什么是异步编程,它和事件驱动及基于回调的编程有何联系,提供了一个良好的背景。因为这些词汇在并发服务器的(非常矛盾的)讨论中很常见。
|
||||
|
||||
让我们从一段 `select` 的手册页面中引用的一句好开始:
|
||||
|
||||
> select,pselect,FD_CLR,FD_ISSET,FD_SET,FD_ZERO - 同步 I/O 处理
|
||||
|
||||
因此 `select` 是 _同步_ 处理。但我刚刚演示了大量代码的例子,使用 `select` 作为 _异步_ 处理服务器的例子。有哪些东西?
|
||||
|
||||
答案是:这取决于你的观查角度。同步常用作阻塞处理,并且对 `select` 的调用实际上是阻塞的。和第 1、2 节中讲到的顺序的、多线程的服务器中对 `send` 和 `recv` 是一样的。因此说 `select` 是 _同步的_ API 是有道理的。可是,服务器的设计却可以是 _异步的_,或是 _基于回调的_,或是 _事件驱动的_,尽管其中有对 `select` 的使用。注意这里的 `on_peer_*` 函数是回调函数;它们永远不会阻塞,并且只有网络事件触发的时候才会被调用。它们可以获得部分数据,并能够在调用过程中保持稳定的状态。
|
||||
|
||||
如果你曾经做过一些 GUI 编程,这些东西对你来说应该很亲切。有个 “事件循环”,常常完全隐藏在框架里,应用的 “业务逻辑” 建立在回调上,这些回调会在各种事件触发后被调用,用户点击鼠标,选择菜单,定时器到时间,数据到达套接字,等等。曾经最常见的编程模型是客户端的 JavaScript,这里面有一堆回调函数,它们在浏览网页时用户的行为被触发。
|
||||
|
||||
### select 的局限
|
||||
|
||||
使用 `select` 作为第一个异步服务器的例子对于说明这个概念很有用,而且由于 `select` 是很常见,可移植的 API。但是它也有一些严重的缺陷,在监视的文件描述符非常大的时候就会出现。
|
||||
|
||||
1. 有限的文件描述符的集合大小。
|
||||
|
||||
2. 糟糕的性能。
|
||||
|
||||
从文件描述符的大小开始。`FD_SETSIZE` 是一个编译期常数,在如今的操作系统中,它的值通常是 1024。它被硬编码在 `glibc` 的头文件里,并且不容易修改。它把 `select` 能够监视的文件描述符的数量限制在 1024 以内。曾有些分支想要写出能够处理上万个并发访问的客户端请求的服务器,这个问题很有现实意义。有一些方法,但是不可移植,也很难用。
|
||||
|
||||
糟糕的性能问题就好解决的多,但是依然非常严重。注意当 `select` 返回的时候,它向调用者提供的信息是 “就绪的” 描述符的个数,还有被修改过的描述符集合。描述符集映射着描述符 就绪/未就绪”,但是并没有提供什么有效的方法去遍历所有就绪的描述符。如果只有一个描述符是就绪的,最坏的情况是调用者需要遍历 _整个集合_ 来找到那个描述符。这在监视的描述符数量比较少的时候还行,但是如果数量变的很大的时候,这种方法弊端就凸显出了 ^[注7][23]。
|
||||
|
||||
由于这些原因,为了写出高性能的并发服务器, `select` 已经不怎么用了。每一个流行的操作系统有独特的不可移植的 API,允许用户写出非常高效的事件循环;像框架这样的高级结构还有高级语言通常在一个可移植的接口中包含这些 API。
|
||||
|
||||
### epoll
|
||||
|
||||
举个例子,来看看 `epoll`,Linux 上的关于高容量 I/O 事件通知问题的解决方案。`epoll` 高效的关键之处在于它与内核更好的协作。不是使用文件描述符,`epoll_wait` 用当前准备好的事件填满一个缓冲区。只有准备好的事件添加到了缓冲区,因此没有必要遍历客户端中当前 _所有_ 监视的文件描述符。这简化了查找就绪的描述符的过程,把空间复杂度从 `select` 中的 O(N) 变为了 O(1)。
|
||||
|
||||
关于 `epoll` API 的完整展示不是这里的目的,网上有很多相关资源。虽然你可能猜到了,我还要写一个不同的并发服务器,这次是用 `epool` 而不是 `select`。完整的示例代码 [在这里][24]。实际上,由于大部分代码和 `用 select 的服务器`相同,所以我只会讲要点,在主循环里使用 `epoll`:
|
||||
|
||||
```
|
||||
struct epoll_event accept_event;
|
||||
accept_event.data.fd = listener_sockfd;
|
||||
accept_event.events = EPOLLIN;
|
||||
if (epoll_ctl(epollfd, EPOLL_CTL_ADD, listener_sockfd, &accept_event) < 0) {
|
||||
perror_die("epoll_ctl EPOLL_CTL_ADD");
|
||||
}
|
||||
|
||||
struct epoll_event* events = calloc(MAXFDS, sizeof(struct epoll_event));
|
||||
if (events == NULL) {
|
||||
die("Unable to allocate memory for epoll_events");
|
||||
}
|
||||
|
||||
while (1) {
|
||||
int nready = epoll_wait(epollfd, events, MAXFDS, -1);
|
||||
for (int i = 0; i < nready; i++) {
|
||||
if (events[i].events & EPOLLERR) {
|
||||
perror_die("epoll_wait returned EPOLLERR");
|
||||
}
|
||||
|
||||
if (events[i].data.fd == listener_sockfd) {
|
||||
// 监听的套接字就绪了;意味着新客户端正在连接。
|
||||
...
|
||||
} else {
|
||||
// A peer socket is ready.
|
||||
if (events[i].events & EPOLLIN) {
|
||||
// 准备好了读取
|
||||
...
|
||||
} else if (events[i].events & EPOLLOUT) {
|
||||
// 准备好了写入
|
||||
...
|
||||
}
|
||||
}
|
||||
}
|
||||
}
|
||||
```
|
||||
|
||||
通过调用 `epoll_ctl` 来配置 `epoll`。这时,配置监听的套接字数量,也就是 `epoll` 监听的描述符的数量。然后分配一个缓冲区,把就绪的事件传给 `epoll` 以供修改。在主循环里对 `epoll_wait` 的调用是魅力所在。它阻塞着,直到某个描述符就绪了(或者超时),返回就绪的描述符数量。但这时,不少盲目地迭代所有监视的集合,我们知道 `epoll_write` 会修改传给它的 `events` 缓冲区,缓冲区中有就绪的事件,从 0 到 `nready-1`,因此我们只需迭代必要的次数。
|
||||
|
||||
要在 `select` 里面重新遍历,有明显的差异:如果在监视着 1000 个描述符,只有两个就绪, `epoll_waits` 返回的是 `nready=2`,然后修改 `events` 缓冲区最前面的两个元素,因此我们只需要“遍历”两个描述符。用 `select` 我们就需要遍历 1000 个描述符,找出哪个是就绪的。因此,在繁忙的服务器上,有许多活跃的套接字时 `epoll` 比 `select` 更加容易扩展。
|
||||
|
||||
剩下的代码很直观,因为我们已经很熟悉 `select 服务器` 了。实际上,`epoll 服务器` 中的所有“业务逻辑”和 `select 服务器` 是一样的,回调构成相同的代码。
|
||||
|
||||
这种相似是通过将事件循环抽象分离到一个库/框架中。我将会详述这些内容,因为很多优秀的程序员曾经也是这样做的。相反,下一篇文章里我们会了解 `libuv`,一个最近出现的更加受欢迎的时间循环抽象层。像 `libuv` 这样的库让我们能够写出并发的异步服务器,并且不用考虑系统调用下繁琐的细节。
|
||||
|
||||
* * *
|
||||
|
||||
|
||||
[注1][1] 我试着在两件事的实际差别中突显自己,一件是做一些网络浏览和阅读,但经常做得头疼。有很多不同的选项,从“他们是一样的东西”到“一个是另一个的子集”,再到“他们是完全不同的东西”。在面临这样主观的观点时,最好是完全放弃这个问题,专注特殊的例子和用例。
|
||||
|
||||
[注2][2] POSIX 表示这可以是 `EAGAIN`,也可以是 `EWOULDBLOCK`,可移植应用应该对这两个都进行检查。
|
||||
|
||||
[注3][3] 和这个系列所有的 C 示例类似,代码中用到了某些助手工具来设置监听套接字。这些工具的完整代码在这个 [仓库][4] 的 `utils` 模块里。
|
||||
|
||||
[注4][5] `select` 不是网络/套接字专用的函数,它可以监视任意的文件描述符,有可能是硬盘文件,管道,终端,套接字或者 Unix 系统中用到的任何文件描述符。这篇文章里,我们主要关注它在套接字方面的应用。
|
||||
|
||||
[注5][6] 有多种方式用多线程来实现事件驱动,我会把它放在稍后的文章中进行讨论。
|
||||
|
||||
[注6][7] 由于各种非实验因素,它 _仍然_ 可以阻塞,即使是在 `select` 说它就绪了之后。因此服务器上打开的所有套接字都被设置成非阻塞模式,如果对 `recv` 或 `send` 的调用返回了 `EAGAIN` 或者 `EWOULDBLOCK`,回调函数就装作没有事件发生。阅读示例代码的注释可以了解更多细节。
|
||||
|
||||
[注7][8] 注意这比该文章前面所讲的异步 polling 例子要稍好一点。polling 需要 _一直_ 发生,而 `select` 实际上会阻塞到有一个或多个套接字准备好读取/写入;`select` 会比一直询问浪费少得多的 CPU 时间。
|
||||
|
||||
--------------------------------------------------------------------------------
|
||||
|
||||
via: https://eli.thegreenplace.net/2017/concurrent-servers-part-3-event-driven/
|
||||
|
||||
作者:[Eli Bendersky][a]
|
||||
译者:[GitFuture](https://github.com/GitFuture)
|
||||
校对:[校对者ID](https://github.com/校对者ID)
|
||||
|
||||
本文由 [LCTT](https://github.com/LCTT/TranslateProject) 原创编译,[Linux中国](https://linux.cn/) 荣誉推出
|
||||
|
||||
[a]:https://eli.thegreenplace.net/pages/about
|
||||
[1]:https://eli.thegreenplace.net/2017/concurrent-servers-part-3-event-driven/#id1
|
||||
[2]:https://eli.thegreenplace.net/2017/concurrent-servers-part-3-event-driven/#id3
|
||||
[3]:https://eli.thegreenplace.net/2017/concurrent-servers-part-3-event-driven/#id4
|
||||
[4]:https://github.com/eliben/code-for-blog/blob/master/2017/async-socket-server/utils.h
|
||||
[5]:https://eli.thegreenplace.net/2017/concurrent-servers-part-3-event-driven/#id5
|
||||
[6]:https://eli.thegreenplace.net/2017/concurrent-servers-part-3-event-driven/#id6
|
||||
[7]:https://eli.thegreenplace.net/2017/concurrent-servers-part-3-event-driven/#id8
|
||||
[8]:https://eli.thegreenplace.net/2017/concurrent-servers-part-3-event-driven/#id9
|
||||
[9]:https://eli.thegreenplace.net/tag/concurrency
|
||||
[10]:https://eli.thegreenplace.net/tag/c-c
|
||||
[11]:http://eli.thegreenplace.net/2017/concurrent-servers-part-1-introduction/
|
||||
[12]:http://eli.thegreenplace.net/2017/concurrent-servers-part-1-introduction/
|
||||
[13]:http://eli.thegreenplace.net/2017/concurrent-servers-part-2-threads/
|
||||
[14]:http://eli.thegreenplace.net/2017/concurrent-servers-part-3-event-driven/
|
||||
[15]:https://eli.thegreenplace.net/2017/concurrent-servers-part-3-event-driven/#id11
|
||||
[16]:https://eli.thegreenplace.net/2017/concurrent-servers-part-3-event-driven/#id12
|
||||
[17]:https://eli.thegreenplace.net/2017/concurrent-servers-part-3-event-driven/#id13
|
||||
[18]:https://github.com/eliben/code-for-blog/blob/master/2017/async-socket-server/select-server.c
|
||||
[19]:https://eli.thegreenplace.net/2017/concurrent-servers-part-3-event-driven/#id14
|
||||
[20]:https://github.com/eliben/code-for-blog/blob/master/2017/async-socket-server/select-server.c
|
||||
[21]:https://eli.thegreenplace.net/2017/concurrent-servers-part-3-event-driven/#id15
|
||||
[22]:http://eli.thegreenplace.net/2017/concurrent-servers-part-2-threads/
|
||||
[23]:https://eli.thegreenplace.net/2017/concurrent-servers-part-3-event-driven/#id16
|
||||
[24]:https://github.com/eliben/code-for-blog/blob/master/2017/async-socket-server/epoll-server.c
|
||||
[25]:https://eli.thegreenplace.net/2017/concurrent-servers-part-3-event-driven/
|
||||
[26]:http://eli.thegreenplace.net/2017/concurrent-servers-part-1-introduction/
|
||||
[27]:http://eli.thegreenplace.net/2017/concurrent-servers-part-2-threads/
|
||||
[28]:https://eli.thegreenplace.net/2017/concurrent-servers-part-3-event-driven/#id10
|
Loading…
Reference in New Issue
Block a user